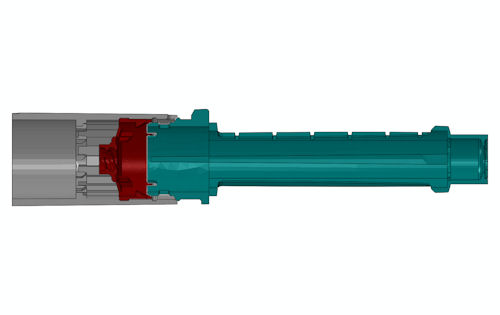
Latest News
July 11, 2013
Many medical conditions can be treated with tablets, but others require injections under the skin for therapeutic drugs to reach the bloodstream. In the case of insulin administration for diabetes treatment, patients need to self-inject the drug daily.
Making those injections easy and safe is of prime importance for Novo Nordisk, the Danish company that has been a world leader in the production of insulin ever since Canadian scientists discovered it in the 1920s. The company innovated beyond standard syringe technology to produce the world’s first patient-friendly self-injection system, the NovoPen, some 25 years ago.
With more than 350 million diabetics worldwide — almost 5% of the global population — and growing, demand for insulin pens will likely remain strong into the foreseeable future. Because effective control of the disease is dependent on consistent use of the drug, these delivery systems need to be portable, easy-to-use, reliable, and even resistant to minor misuse by patients.
Small Device, Big Design Task
An insulin pen may be small, but it is a precision instrument with a number of complex parts that must work in perfect tandem. Some pens are durable, containing a replaceable drug cartridge, while other disposable ones come pre-filled with the drug.
Injection typically involves twisting a short needle onto the pen, turning a dial to the required dose, and pushing a button to deliver the medication under the skin. After a given number of doses are injected, the cartridge is exchanged for a new one (with a durable device) or discarded (with disposable pens).
![]() | ![]() |
Figs. 1A and 1B. At left is a CAD image of the components of a diabetes pen. Grey and red cylindrical parts are snapped onto the green. At right is an injection-molded ratchet component from a medical device used by Novo Nordisk for a snap-fit benchmark study. |
Audible clicks that occur at key stages of this procedure reassure the patient that he or she is engaging the device correctly at each step. It looks pretty easy (a one-minute video of a woman checking her blood sugar and then using a NovoPen to inject insulin is available at NovoNordisk.com/press/broadcastroom/default.asp). But every one of those reassuring clicks represents a challenge that has been overcome by the engineers who created the pens. So do the clicks the patient never hears: those that occur as the pen parts are assembled in the factory before use.
“Parts that click into place with ‘snap fit’ instead of screw connectors are very efficient to assemble within mass production,” says Torben Strom Hansen, Ph.D., principal scientist in the Device R&D division of Novo Nordisk, near Copenhagen, Denmark. “Snap fit is the commonly used way to connect parts in our device mechanisms, and it also signals reliability when the internal components have optimal connections that don’t rattle. It’s very efficient when designed correctly.”
![]() | ![]() |
Figs. 2A and 2B. Polymer material response from cyclic loading and unloading (left), and multiple cycles repeatedly stressing the material by increasing the strain for each cycle (right). |
Getting those designs correct from the start is the task that Hansen and his Mechanical Analysis team focus on in close collaboration with Novo Nordisk’s mechanical designers. “Even though an injection pen is not that big, there are a lot of fine details in its design,” he says. Whatever the configuration of device, the plastic-polymer components must withstand the rigors of both manufacturing and patient use, performing as required at different temperatures and loads.
Teaming up to Model Polymer Behavior
To ensure the integrity of their designs, Hansen and his team in the Device Simulation department rely on computer simulation with Abaqus finite element analysis (FEA) software from Dassault Systèmes’ SIMULIA brand.
“More than a decade ago, my colleagues and I explored a number of commercial software codes,” he says. “We chose Abaqus because it was a well-integrated solution that provided both implicit and explicit capabilities, and could model the non-linear behavior of the fine details in our designs correctly, including the high number of interfaces in contact.”
![]() | ![]() |
Figs. 3A and 3B. Component loading (left) and resulting test reaction force vs. displacement comparison (right). The ratchet arm at left is being deliberately overstrained to see how well the material model demonstrates the large strain cyclic behavior. |
Over time, the group’s device models have become more refined, sophisticated and computationally demanding. The SIMULIA Polymer Customer Review Team (PCRT) has worked closely with Novo Nordisk all along to provide updated enhancements in Abaqus that enable the company to model and predict the complexities of polymer behavior with increasing accuracy and efficiency. The PCRT includes members from the automotive, high-tech, life sciences and consumer goods industries.
The Snap-fit Challenge
A recent focus on snap fits in insulin pens demonstrates the challenges the team has faced when modeling polymers. “We concentrated on snap fits because they demonstrate almost ideal cyclic loading, with parts repeatedly loading and unloading from single to multiple cycles,” says Hansen.
During such cycles, the viscous nature of the thermoplastic material determines how the bouncing back to “normal” occurs. Prediction through analysis of such time-dependent behavior is key to the device development process.
![]() Fig. 4. FEA demonstrates the ultimate straining of the snap fit on the device components during assembly. |
Because devices can be subjected to different environments, including elevated temperatures, the function of the device must be as unaffected as possible by such changes; it must always comply with the specification even though the material properties of the components vary. Even just sitting on a pharmacy shelf or in the medicine cabinet, polymer materials are prone to creep and relaxation over time at rates that can vary with the temperature. Some polymers are also more complex than others: Those used in durable devices may contain carbon or glass fillers that show anisotropic behavior, which can be hard to predict.
“Modeling these diverse material characteristics, as well as the behavior of the polymer as the load induces larger strains closer to yield, is difficult,” says Hansen. “To predict such viscoelasticity precisely, we needed a more refined model that goes beyond a mainstream elastic-plastic approach.”
Modeling Material That’s Constantly Changing
The team is now using the “parallel rheological framework” methodology available in Abaqus to model polymer non-linear viscoelasticity with greater accuracy than ever before. The framework makes use of an arbitrary number of viscoelastic networks and an elastic equilibrium network to create a specific, non-linear viscoelastic model. It is used to predict and track changes in the internal structural networks of a polymer as the material responds to repeated cyclic loads during snap fit. The material parameters in the FEA model are updated at each time step to reflect the new, altered state of the polymer. Because every type of polymer shows a different response to temperature, load, etc., the team continues to explore ways to identify the material characteristics of different polymer networks.
![]() Fig. 5. At left, the final snap deformation model in Abaqus FEA (using a parallel framework to capture the changing behavior of the polymer material under cyclic loading) shows a more accurate plastic deformation of 0.12mm. The earlier elastic-plastic model on the right overpredicted that deformation would be 0.66mm. |
Not only are such advanced models useful to designers fine-tuning the latest pen configuration, the data can help inform manufacturing processes in the factory.
“We have a process-simulating capability, through Moldflow, for which Abaqus has an interface. This allows us to input the stress fields that result from the molding process right into our models,” says Hansen. “As a result, we have greater insight into our manufacturing process and are more able to design parts that have a very low level of residual stresses in critical regions.
“SIMULIA is working closely with us to provide capabilities we need,” Hansen adds. “Having material models incorporating time-dependent viscous behavior is very important for our work, and we’re now able to simulate both creep and relaxation with Abaqus. We are investigating how well the model will adapt to different kinds of thermoplastics, which may require different networks. Calibration will be key going forward.”
More Info
Subscribe to our FREE magazine,
FREE email newsletters or both!Latest News
About the Author
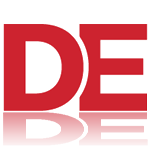
DE’s editors contribute news and new product announcements to Digital Engineering.
Press releases may be sent to them via [email protected].

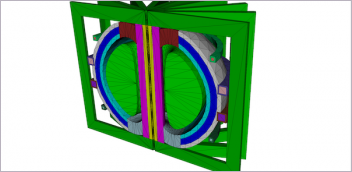
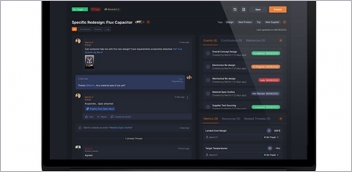
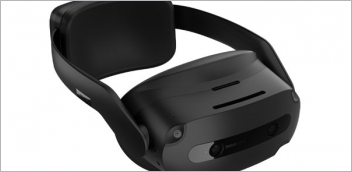