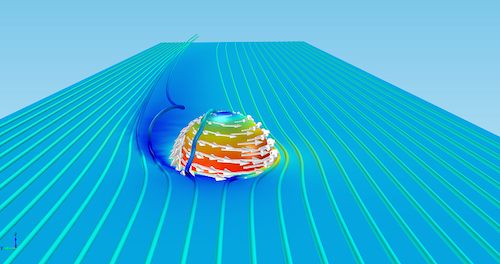
The velocity and pressure fields on the soccer ball as illustrated with CFD analysis. Image courtesy of COMSOL, Inc.
Latest News
June 13, 2014
With the World Cup 2014 games kicking off this week, the entire world is transfixed with all things soccer (or football-related, depending on where you hail). As we get ready to cheer on our favorite teams and marvel at the unbridled athleticism, it’s worth a look at the serious science and engineering at play behind all of that fancy footwork.
Physics plays a starring role in any sport, and soccer is no exception. The Magnus effect, a principle that explains the side-force on a sphere that is both rotating and moving forward, is used extensively to analyze the World Cup match balls used in this and previous years’ games.
Ed Fontes of CAE provider COMSOL explained on the company blog how the Magnus effect can be applied to understand the trajectory of a soccer ball. Here are some excerpts from his explanation.
In instances where there is no spin on the ball, a Karman vortex street is formed behind it, which is then subjected to force fluctuations with the detachment of vortices behind it. The wakes that are formed behind the ball both increase the drag and contribute to swerving, such as that of a beach ball.
“As the rotation velocity increases, the stagnation points on the ball move together and eventually outside its surface,” Fontes explained in the post. “At this point, the velocity due to the rotation of the ball is perfectly balanced by the ball’s forward movement. Here, the flight of the ball is stabilized and is much more predictable — at least for the player shooting the ball or the goalie.”
Additionally, a pressure difference is built up between the two sides of the ball because of the difference in speed and shear between the two sides. This results in a force that sucks the ball toward the side, where the air velocity is higher. That is the Magnus force acting on the ball, allowing David Beckham and other players to “bend it” around defenders. An increased lift coefficient with the spin further reflects this.
Another principle that sheds light on soccer ball trajectory is the high drag coefficients for laminar flow. These are caused by boundary layer separation forming a low-pressure wake that slows down the ball in the laminar flow regime. For flights of higher velocities, the boundary layer becomes turbulent ahead of the separation point and continues to be attached further downstream on the rear side of the ball before the flow reverses. As a result, there is a narrower wake and correspondingly lower drag coefficient. This is referred to as the drag crisis.
The Magnus effect and the drag crisis have had a big influence on some of the newer and more revolutionary soccer ball designs. Consider the Adidas Jabulani, released for the FIFA World Cup 2010 in South Africa. While the traditional soccer ball has 32 panels, comprising 20 regular hexagonal and 12 regular pentagonal panels, the Jabulani, in comparison, had eight panels. The design compensated for the reduced number of the seams by adding grooves that roughened the surface. However, the aerodynamic properties of the Jabulani were quite different from the traditional ball.
Because the panels and seams were fewer and shallower on the Jabulani compared to those on a traditional ball, the laminar flow region with high drag coefficients was increased, while decreasing the drag coefficients at higher speeds. The relatively large width of the Jabulani’s laminar region made it behave like a beach ball for a wider velocity range—a fact that was not lost on goalies, who complained vigorously about its surprising path. The way the ball’s pattern interacts with the wind can also promote those sudden direction changes that account for the “knuckleball” effect in baseball.
The official ball of this year’s FIFA World Cup 2014 games in Brazil — the new Adidas Brazuca — sports six panels, while the total length of seams is comparable to a traditional ball. However, the depth of the seams is larger than that of the Jabulani. As Fontes illustrates, because of that design, the drag coefficient as a function of the Reynolds number for the Brazuca is more similar to a traditional soccer ball. As a result, it is expected to have a stable flight over a wider range of velocity.
These principles are manifest in the characteristic game style of popular players. For instance, Portugal’s Cristiano Ronaldo hits a ball hard and consistently without spin, putting it on a straight path far from the goal. In contrast, players like Beckham and Diego Maradona’s trademark ability to repeatedly place a 30-meter cross within a radius of a half a meter from the target can be chalked up to the stable curl of a spinning ball, courtesy of the Magnus effect.
Next time you witness one of those incredible curved goals, keep the Magnus effect in mind. In this video clip, players such as Lionel Messi, Ronaldo, Zlatan Ibrahimovic, Ronaldinho, Beckham and many others make use of this effect to cheat the goalkeepers.
In other science-related World Cup news, the first official kick of the games was made not by a star player banking on the Magnus effect, but rather by Juliano Pinto, a 29-year-old paraplegic. With the help of a mind-controlled robotic exoskeleton, Pinto kicked the official ball a short distance in Corinthians Arena in Sao Paulo, Brazil, along a mat nearby the touchline.
The robotic exoskeleton was created by a team of 150 researchers, led by Miguel Nicolelis, a leading researcher in the field of brain-machine interfaces with Duke University, and supported by the Brazilian government and Sanjay Joshi, professor of mechanical and aerospace engineering at the University of California, Davis. The FIFA World Cup kickoff was part of an outward reach to showcase the Walk Again Project, which is tasked with developing a thought-controlled robotic exoskeleton for disabled people for everyday use.
The Walk Again exoskeleton employs a cap of electrodes and a series of control systems to pick up signals directly from the wearer’s brain as well as muscle signals and transform them into action. Learn more about the exoskeleton from this University of California, Davis video:
Subscribe to our FREE magazine,
FREE email newsletters or both!Latest News
About the Author
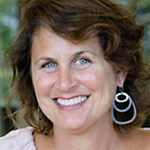
Beth Stackpole is a contributing editor to Digital Engineering. Send e-mail about this article to [email protected].
Follow DERelated Topics

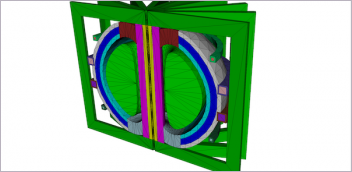
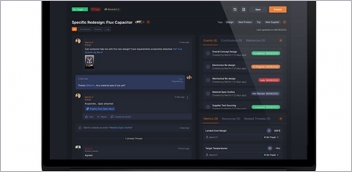
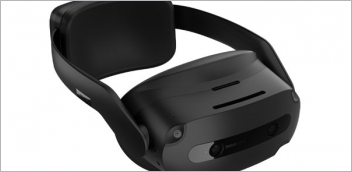