
Using Project Falcon’s 3D visualization capabilities, the team was able to visually communicate the results of the CFD airflow analysis. Image Courtesy of Autodesk
Latest News
December 3, 2013
What do one of the windiest professional football stadiums and a rare breed of flying snake have in common? They are both quirky examples of putting CAE to the test. More importantly, however, both offer some important takeaways for engineers looking to promote simulation studies far earlier in the design process.
Let’s start with the simulation work done in conjunction with the Pittsburgh Steelers’ Heinz Field. Known for killer winds that blow into the stadium and across the field in a variety of crazy patterns, the field is notoriously hard on kickers. Autodesk, with an assist from a Penn State University student and Autodesk summer intern Matt Wilson, decided to apply the company’s technology to explore whether there was any scientific evidence to support the league’s long-standing complaints about the challenging kick-off environment.
Using Autodesk’s suite of simulation tools, Wilson modeled the stadium as well as the particular location’s airflow patterns. He began by using Autodesk’s handheld ForceEffect Flow iPad app to do a simple 2D flow of the stadium and to determine whether there was any foundation for a potential problem, said John Twerdok, Autodesk’s senior business line manager. Once the 2D model illustrated there was just cause to pursue deeper exploration, Wilson created a full 3D macro model of the stadium and the flow before finalizing his experiment with a full 3D CFD analysis, which incorporated Heinz Field’s weather data. For this last step, Wilson employed Project Falcon, Autodesk’s cloud-based CFD prototype service. Project Falcon will be released in mid-December as a production-ready offering called Design Flows.
More than solving any real engineering issue in the construction of Heinz Stadium, the exercise was really a look at how to properly apply simulation tools. “This was really a lesson in the progression of how to use the tools,” Twerdok said. “It’s best to start with the simplest tool out there and then if you see a problem, create the more progressive and complex models and invest more time in the simulation.”
Yet that’s not how most engineering departments attack the problem. “What we often see with users of simulation, especially novices, is they jump in and model everything, but because they don’t know what they are looking for, they end up with something too unwieldy,” he explained. “A very coarse simulation done early in the process could have more impact than doing a more detailed one at the end.”
The Heinz Stadium project also underscored how important visualization is to helping communicate simulation results, particularly to those outside of engineering. “I could have had a lot of numbers, but that wouldn’t matter much,” he said. “The 3D visual images could be put in front of those designing the stadium and give them an idea of the issue and what’s happening.”
Snakes Take Flight
Now what about the idea of using simulation, specifically, GPU-accelerated virtual wind-tunnel simulation, to study the flight patterns of flying snakes? That’s exactly what Lorena Barba, associate professor of mechanical and aerospace engineering at George Washington University in Washington, DC, is doing to understand one of Nature’s mysterious creatures, the Chrysopelea.
Commonly found in Southeast Asia and India, the Chrysopelea has evolved to do something other snakes can only dream of—that is fly. Well, technically, they glide, launching themselves into the air from tall tree branches and gliding down with graceful undulating movements. But how does a snake, without the wingspans of birds, create lift and sustain it?
“These snakes are quite small—in fact, usually not more than a meter long, maybe 1.2 meters,” said Barba. “You can study them in the field, but it’s quite difficult to get the details of the flow of aerodynamics from a snake in midflight. Naturally, it would also be difficult to put a live snake inside a wind tunnel.”
Barba’s collaborator, Jake Socha, an assistant professor of Engineering Science and Mechanics at Virginia Tech, has been studying the very same creatures and has conducted a wind tunnel test that employs a 3D-printed cross-section of the snake’s body.
“[Socha and his team] did this to measure the forces,” said Barba. “They also use DPIV (digital particle image velocimetry) to visualize the flow. That gives you some idea of the lift and drag, but it’ not possible to get details of the flow because the resolution is not as fine.”
Barba and her researchers decided to migrate to computer simulation 1) to see whether it produces the same characteristics seen in DPIV and 2) to explain the mysterious bump in the snake’s lift they’ve observed.
Currently, Barba and her team run simulations on 2D cross-sections of the snake’s body. They use immersed boundary method, “which is particularly suited to deal with moving bodies,” Barba explained. Even in 2D, the simulation exercise involves about four million mesh points. On a workstation with a single NVIDIA Tesla GPU, average jobs run approximately eight to 10 hours. (The shortest runs are about two hours; the longest runs take up to three days.) The job has to run long enough so that the researchers can collect a time signature with reliable lift and drag coefficients.
Reusing the same algorithms developed originally for aviation to study animal flight is a relatively recent trend, Barba pointed out. “It’s rather difficult because the code is not directly applicable to small-scale flows, like those from insects. [Animal flight] is very unsteady, constantly generating little eddies. The wings are constantly moving and flapping. That’s not what happens in aeronautical applications.” Therefore, Barba and her students used a custom-developed code, written from scratch.
“Doing a fully resolved 3D CFD simulation that gives you the correct physics is still quite difficult, and requires lots of computing power,” said Barba. “But we’re leading to that.” Currently, Barba has students working on parallelizing the code for snake-glide simulation. That would allow them to take advantage of the GPU’s parallel-processing architecture—a prelude to running a full 3D simulation of the snake’s flight pattern.
Barba is frequently asked how her work might benefit other commercial aerospace or automotive projects, for example in developing microair vehicles. “I can tell you that knowledge obtained from studying animal locomotion often leads to applications in unexpected situations,” she said, “like the study of schooling fish, for example, somehow helping in the efficient placement of wind turbines in a wind farm.”
Could engineers use her research to produce a flying robot that moves like the snakes? Perhaps, but that’s a straightforward application that everyone can foresee. Barba is much more interested in unexpected applications that might crop up in an entirely unrelated field. For example, someone could engineer an energy-harvesting device that mimics the flying snake’s slow-turning movements. At the moment, she can only speculate.
Check out this video on Jake Socha’s TEDx talk on use of CFD to study flying snakes. And thanks to my colleague Kenneth Wong who contributed the reporting on the flying snakes CAE analysis.
Subscribe to our FREE magazine,
FREE email newsletters or both!Latest News
About the Author
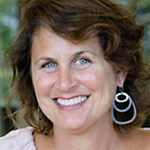
Beth Stackpole is a contributing editor to Digital Engineering. Send e-mail about this article to [email protected].
Follow DE
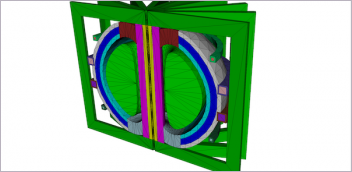
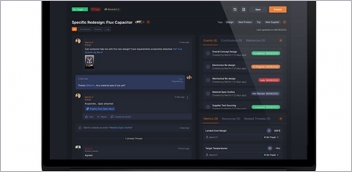
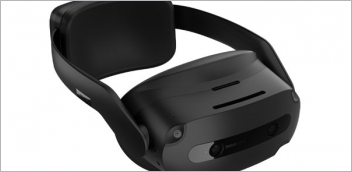